Quantum Numbers,
Atomic Orbitals, and
Electron Configurations
- The atomic number is the number of protons in the nucleus of an atom. The number of protons define the identity of an element (i.e., an element with 6 protons is a carbon atom, no matter how many neutrons may be present). The number of protons determines how many electrons surround the nucleus, and it is the arrangement of these electrons that.
- Hypernyms ('atomic number 2' is a kind of.): chemical element; element(any of the more than 100 known substances (of which 92 occur naturally) that cannot be separated into simpler substances and that singly or in combination constitute all matter).
Contents:
Quantum Numbers and Atomic Orbitals
1. Principal Quantum Number (n)
2.Angular Momentum (Secondary, Azimunthal) Quantum Number (l)
3.Magnetic Quantum Number (ml)
4.Spin Quantum Number (ms)
Table of Allowed Quantum Numbers
Writing Electron Configurations
Properties of Monatomic Ions
References
Quantum Numbers and Atomic Orbitals
By solving the Schrödinger equation (Hy = Ey), we obtain a set of mathematical equations, called wave functions (y), which describe the probability of finding electrons at certain energy levels within an atom.
A wave function for an electron in an atom is called an atomic orbital; this atomic orbital describes a region of space in which there is a high probability of finding the electron. Energy changes within an atom are the result of an electron changing from a wave pattern with one energy to a wave pattern with a different energy (usually accompanied by the absorption or emission of a photon of light).
The sum of the atomic number Z and the number of neutrons N gives the mass number A of an atom. Since protons and neutrons have approximately the same mass (and the mass of the electrons is negligible for many purposes) and the mass defect of nucleon binding is always small compared to the nucleon mass, the atomic mass of any atom, when expressed in unified atomic mass units (making a quantity. Atomic number, the number of a chemical element in the periodic system, whereby the elements are arranged in order of increasing number of protons in the nucleus. Accordingly, the number of protons, which is always equal to the number of electrons in a neutral atom, is also the atomic number. A chemical element with an atomic number of two. In its most abundant form it consists of a nucleus of two protons and two neutrons, around which orbit two electrons. This nucleus, i.e. The positively charged helium ion, is exceptionally stable; it is called an alpha particle.
Each electron in an atom is described by four different quantum numbers. The first three (n, l, ml) specify the particular orbital of interest, and the fourth (ms) specifies how many electrons can occupy that orbital.
- Principal Quantum Number (n): n = 1, 2, 3, …, ∞
Specifies the energy of an electron and the size of the orbital (the distance from the nucleus of the peak in a radial probability distribution plot). All orbitals that have the same value of n are said to be in the same shell (level). For a hydrogen atom with n=1, the electron is in its ground state; if the electron is in the n=2 orbital, it is in an excited state. The total number of orbitals for a given n value is n2.
Atomic Number For Helium
- Angular Momentum (Secondary, Azimunthal) Quantum Number (l): l = 0, ..., n-1.
Specifies the shape of an orbital with a particular principal quantum number. The secondary quantum number divides the shells into smaller groups of orbitals called subshells (sublevels). Usually, a letter code is used to identify l to avoid confusion with n:
l | 0 | 1 | 2 | 3 | 4 | 5 | ... |
Letter | s | p | d | f | g | h | ... |
The subshell with n=2 and l=1 is the 2p subshell; if n=3 and l=0, it is the 3s subshell, and so on. The value of l also has a slight effect on the energy of the subshell; the energy of the subshell increases with l (s < p < d < f).
- Magnetic Quantum Number (ml): ml = -l, ..., 0, ..., +l.
Specifies the orientation in space of an orbital of a given energy (n) and shape (l). This number divides the subshell into individual orbitals which hold the electrons; there are 2l+1 orbitals in each subshell. Thus the s subshell has only one orbital, the p subshell has three orbitals, and so on.
- Spin Quantum Number (ms): ms = +½ or -½.
Specifies the orientation of the spin axis of an electron. An electron can spin in only one of two directions (sometimes called up and down).
The Pauli exclusion principle (Wolfgang Pauli, Nobel Prize 1945) states that no two electrons in the same atom can have identical values for all four of their quantum numbers. What this means is that no more than two electrons can occupy the same orbital, and that two electrons in the same orbital must have opposite spins.
Because an electron spins, it creates a magnetic field, which can be oriented in one of two directions. For two electrons in the same orbital, the spins must be opposite to each other; the spins are said to be paired. These substances are not attracted to magnets and are said to be diamagnetic. Atoms with more electrons that spin in one direction than another contain unpaired electrons. These substances are weakly attracted to magnets and are said to be paramagnetic.
Table of Allowed Quantum Numbers
n | l | ml | Number of orbitals | Orbital Name | Number of electrons |
1 | 0 | 0 | 1 | 1s | 2 |
2 | 0 | 0 | 1 | 2s | 2 |
1 | -1, 0, +1 | 3 | 2p | 6 | |
3 | 0 | 0 | 1 | 3s | 2 |
1 | -1, 0, +1 | 3 | 3p | 6 | |
2 | -2, -1, 0, +1, +2 | 5 | 3d | 10 | |
4 | 0 | 0 | 1 | 4s | 2 |
1 | -1, 0, +1 | 3 | 4p | 6 | |
2 | -2, -1, 0, +1, +2 | 5 | 4d | 10 | |
3 | -3, -2, -1, 0, +1, +2, +3 | 7 | 4f | 14 |
Writing Electron Configurations
The distribution of electrons among the orbitals of an atom is called the electron configuration. The electrons are filled in according to a scheme known as the Aufbau principle ('building-up'), which corresponds (for the most part) to increasing energy of the subshells:
1s, 2s, 2p, 3s, 3p, 4s, 3d, 4p, 5s, 4d, 5p, 6s, 4f, 5d, 6p, 7s, 5f
It is not necessary to memorize this listing, because the order in which the electrons are filled in can be read from the periodic table in the following fashion:
Or, to summarize:
In electron configurations, write in the orbitals that are occupied by electrons, followed by a superscript to indicate how many electrons are in the set of orbitals (e.g., H 1s1)
Another way to indicate the placement of electrons is an orbital diagram, in which each orbital is represented by a square (or circle), and the electrons as arrows pointing up or down (indicating the electron spin). When electrons are placed in a set of orbitals of equal energy, they are spread out as much as possible to give as few paired electrons as possible (Hund's rule).

examples will be added at a later date
In a ground state configuration, all of the electrons are in as low an energy level as it is possible for them to be. When an electron absorbs energy, it occupies a higher energy orbital, and is said to be in an excited state.
Properties of Monatomic Ions
The electrons in the outermost shell (the ones with the highest value of n) are the most energetic, and are the ones which are exposed to other atoms. This shell is known as the valence shell. The inner, core electrons (inner shell) do not usually play a role in chemical bonding.
Elements with similar properties generally have similar outer shell configurations. For instance, we already know that the alkali metals (Group I) always form ions with a +1 charge; the 'extra' s1 electron is the one that's lost:
IA | Li | 1s22s1 | Li+ | 1s2 |
Na | 1s22s22p63s1 | Na+ | 1s22s22p6 | |
K | 1s22s22p63s23p64s1 | K+ | 1s22s22p63s23p6 |
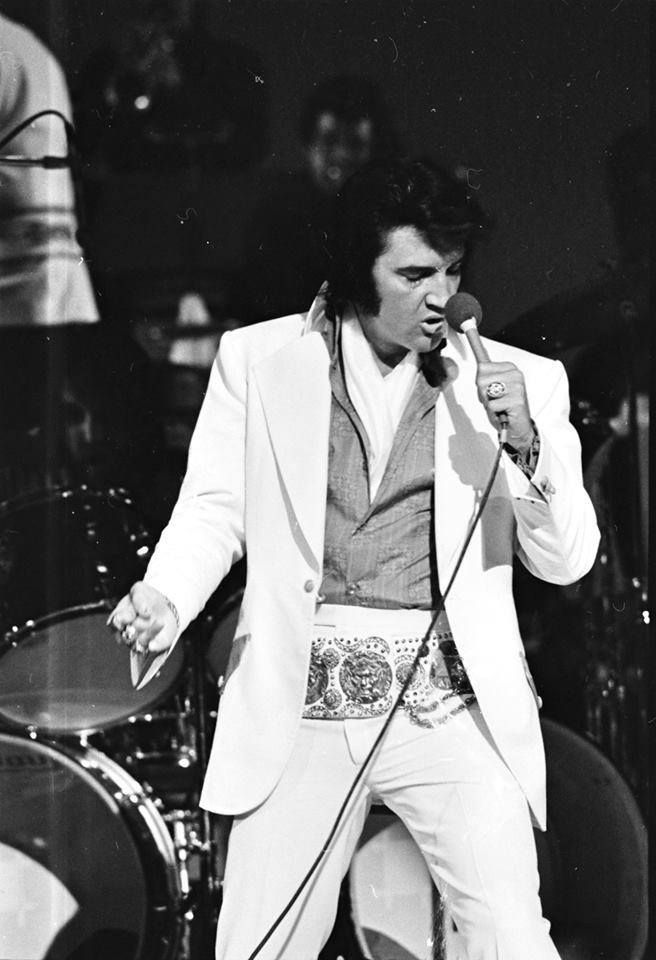
The next shell down is now the outermost shell, which is now full — meaning there is very little tendency to gain or lose more electrons. The ion's electron configuration is the same as the nearest noble gas — the ion is said to be isoelectronic with the nearest noble gas. Atoms 'prefer' to have a filled outermost shell because this is more electronically stable.
- The Group IIA and IIIA metals also tend to lose all of their valence electrons to form cations.
IIA | Be | 1s22s2 | Be2+ | 1s2 |
Mg | 1s22s22p63s2 | Mg2+ | 1s22s22p6 | |
IIIA | Al | 1s22s22p63s23p1 | Al3+ | 1s22s22p6 |
- The Group IV and V metals can lose either the electrons from the p subshell, or from both the s and p subshells, thus attaining a pseudo-noble gas configuration.
IVA | Sn | [Kr]4d105s25p2 | Sn2+ | [Kr]4d105s2 |
Sn4+ | [Kr]4d10 | |||
Pb | [Xe]4f145d106s26p2 | Pb2+ | [Xe]4f145d106s2 | |
Pb4+ | [Xe]4f145d10 | |||
VA | Bi | [Xe]4f145d106s26p3 | Bi3+ | [Xe]4f145d106s2 |
Bi5+ | [Xe]4f145d10 |
- The Group IV - VII non-metals gain electrons until their valence shells are full (8 electrons).
IVA | C | 1s22s22p2 | C4- | 1s22s22p6 |
VA | N | 1s22s22p3 | N3- | 1s22s22p6 |
VIA | O | 1s22s22p4 | O2- | 1s22s22p6 |
VIIA | F | 1s22s22p5 | F- | 1s22s22p6 |
- The Group VIII noble gases already possess a full outer shell, so they have no tendency to form ions.
- Transition metals (B-group) usually form +2 charges from losing the valence s electrons, but can also lose electrons from the highest d level to form other charges.
B-group | Fe | 1s22s22p63s23p63d64s2 | Fe2+ | 1s22s22p63s23p63d6 |
Fe3+ | 1s22s22p63s23p63d5 |

References
Martin S. Silberberg, Chemistry: The Molecular Nature of Matter and Change, 2nd ed. Boston: McGraw-Hill, 2000, p. 277-284, 293-307.
The atomic number or proton number (symbol Z) of a chemical element is the number of protons found in the nucleus of every atom of that element. The atomic number uniquely identifies a chemical element. It is identical to the charge number of the nucleus. In an uncharged atom, the atomic number is also equal to the number of electrons.
The sum of the atomic number Z and the number of neutronsN gives the mass numberA of an atom. Since protons and neutrons have approximately the same mass (and the mass of the electrons is negligible for many purposes) and the mass defect of nucleon binding is always small compared to the nucleon mass, the atomic mass of any atom, when expressed in unified atomic mass units (making a quantity called the 'relative isotopic mass'), is within 1% of the whole number A.
Atoms with the same atomic number but different neutron numbers, and hence different mass numbers, are known as isotopes. A little more than three-quarters of naturally occurring elements exist as a mixture of isotopes (see monoisotopic elements), and the average isotopic mass of an isotopic mixture for an element (called the relative atomic mass) in a defined environment on Earth, determines the element's standard atomic weight. Historically, it was these atomic weights of elements (in comparison to hydrogen) that were the quantities measurable by chemists in the 19th century.
The conventional symbol Z comes from the German word Zahl meaning number, which, before the modern synthesis of ideas from chemistry and physics, merely denoted an element's numerical place in the periodic table, whose order is approximately, but not completely, consistent with the order of the elements by atomic weights. Only after 1915, with the suggestion and evidence that this Z number was also the nuclear charge and a physical characteristic of atoms, did the word Atomzahl (and its English equivalent atomic number) come into common use in this context.
History[edit]
The periodic table and a natural number for each element[edit]
Loosely speaking, the existence or construction of a periodic table of elements creates an ordering of the elements, and so they can be numbered in order.
Dmitri Mendeleev claimed that he arranged his first periodic tables (first published on March 6, 1869) in order of atomic weight ('Atomgewicht').[1] However, in consideration of the elements' observed chemical properties, he changed the order slightly and placed tellurium (atomic weight 127.6) ahead of iodine (atomic weight 126.9).[1][2] This placement is consistent with the modern practice of ordering the elements by proton number, Z, but that number was not known or suspected at the time.
A simple numbering based on periodic table position was never entirely satisfactory, however. Besides the case of iodine and tellurium, later several other pairs of elements (such as argon and potassium, cobalt and nickel) were known to have nearly identical or reversed atomic weights, thus requiring their placement in the periodic table to be determined by their chemical properties. However the gradual identification of more and more chemically similar lanthanide elements, whose atomic number was not obvious, led to inconsistency and uncertainty in the periodic numbering of elements at least from lutetium (element 71) onward (hafnium was not known at this time).
The Rutherford-Bohr model and van den Broek[edit]
In 1911, Ernest Rutherford gave a model of the atom in which a central nucleus held most of the atom's mass and a positive charge which, in units of the electron's charge, was to be approximately equal to half of the atom's atomic weight, expressed in numbers of hydrogen atoms. This central charge would thus be approximately half the atomic weight (though it was almost 25% different from the atomic number of gold (Z = 79, A = 197), the single element from which Rutherford made his guess). Nevertheless, in spite of Rutherford's estimation that gold had a central charge of about 100 (but was element Z = 79 on the periodic table), a month after Rutherford's paper appeared, Antonius van den Broek first formally suggested that the central charge and number of electrons in an atom was exactly equal to its place in the periodic table (also known as element number, atomic number, and symbolized Z). This proved eventually to be the case.
Moseley's 1913 experiment[edit]
The experimental position improved dramatically after research by Henry Moseley in 1913.[3] Moseley, after discussions with Bohr who was at the same lab (and who had used Van den Broek's hypothesis in his Bohr model of the atom), decided to test Van den Broek's and Bohr's hypothesis directly, by seeing if spectral lines emitted from excited atoms fitted the Bohr theory's postulation that the frequency of the spectral lines be proportional to the square of Z.
To do this, Moseley measured the wavelengths of the innermost photon transitions (K and L lines) produced by the elements from aluminum (Z = 13) to gold (Z = 79) used as a series of movable anodic targets inside an x-ray tube.[4] The square root of the frequency of these photons (x-rays) increased from one target to the next in an arithmetic progression. This led to the conclusion (Moseley's law) that the atomic number does closely correspond (with an offset of one unit for K-lines, in Moseley's work) to the calculated electric charge of the nucleus, i.e. the element number Z. Among other things, Moseley demonstrated that the lanthanide series (from lanthanum to lutetium inclusive) must have 15 members—no fewer and no more—which was far from obvious from known chemistry at that time.
Missing elements[edit]
After Moseley's death in 1915, the atomic numbers of all known elements from hydrogen to uranium (Z = 92) were examined by his method. There were seven elements (with Z < 92) which were not found and therefore identified as still undiscovered, corresponding to atomic numbers 43, 61, 72, 75, 85, 87 and 91.[5] From 1918 to 1947, all seven of these missing elements were discovered.[6] By this time, the first four transuranium elements had also been discovered, so that the periodic table was complete with no gaps as far as curium (Z = 96).
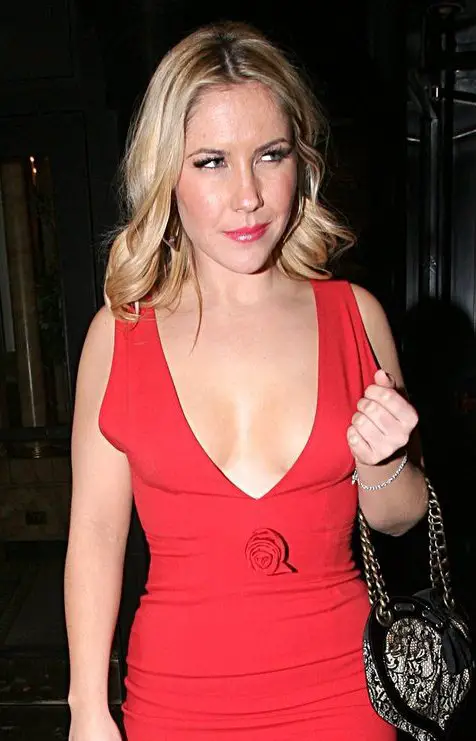
The proton and the idea of nuclear electrons[edit]
In 1915, the reason for nuclear charge being quantized in units of Z, which were now recognized to be the same as the element number, was not understood. An old idea called Prout's hypothesis had postulated that the elements were all made of residues (or 'protyles') of the lightest element hydrogen, which in the Bohr-Rutherford model had a single electron and a nuclear charge of one. However, as early as 1907, Rutherford and Thomas Royds had shown that alpha particles, which had a charge of +2, were the nuclei of helium atoms, which had a mass four times that of hydrogen, not two times. If Prout's hypothesis were true, something had to be neutralizing some of the charge of the hydrogen nuclei present in the nuclei of heavier atoms.
In 1917, Rutherford succeeded in generating hydrogen nuclei from a nuclear reaction between alpha particles and nitrogen gas,[7] and believed he had proven Prout's law. He called the new heavy nuclear particles protons in 1920 (alternate names being proutons and protyles). It had been immediately apparent from the work of Moseley that the nuclei of heavy atoms have more than twice as much mass as would be expected from their being made of hydrogen nuclei, and thus there was required a hypothesis for the neutralization of the extra protons presumed present in all heavy nuclei. A helium nucleus was presumed to be composed of four protons plus two 'nuclear electrons' (electrons bound inside the nucleus) to cancel two of the charges. At the other end of the periodic table, a nucleus of gold with a mass 197 times that of hydrogen was thought to contain 118 nuclear electrons in the nucleus to give it a residual charge of +79, consistent with its atomic number.
The discovery of the neutron makes Z the proton number[edit]
All consideration of nuclear electrons ended with James Chadwick's discovery of the neutron in 1932. An atom of gold now was seen as containing 118 neutrons rather than 118 nuclear electrons, and its positive charge now was realized to come entirely from a content of 79 protons. After 1932, therefore, an element's atomic number Z was also realized to be identical to the proton number of its nuclei.
The symbol of Z[edit]
Atomic Number 206
The conventional symbol Z possibly comes from the German word Atomzahl (atomic number).[8] However, prior to 1915, the word Zahl (simply number) was used for an element's assigned number in the periodic table.
Chemical properties[edit]
Each element has a specific set of chemical properties as a consequence of the number of electrons present in the neutral atom, which is Z (the atomic number). The configuration of these electrons follows from the principles of quantum mechanics. The number of electrons in each element's electron shells, particularly the outermost valence shell, is the primary factor in determining its chemical bonding behavior. Hence, it is the atomic number alone that determines the chemical properties of an element; and it is for this reason that an element can be defined as consisting of any mixture of atoms with a given atomic number.
New elements[edit]
The quest for new elements is usually described using atomic numbers. As of 2021, all elements with atomic numbers 1 to 118 have been observed. Synthesis of new elements is accomplished by bombarding target atoms of heavy elements with ions, such that the sum of the atomic numbers of the target and ion elements equals the atomic number of the element being created. In general, the half-life of a nuclide becomes shorter as atomic number increases, though undiscovered nuclides with certain 'magic' numbers of protons and neutrons may have relatively longer half-lives and comprise an island of stability.
Atomic Number 26
See also[edit]
Look up atomic number in Wiktionary, the free dictionary. |
Atomic Number 26
References[edit]
- ^ abThe Periodic Table of Elements, American Institute of Physics
- ^The Development of the Periodic Table, Royal Society of Chemistry
- ^Ordering the Elements in the Periodic Table, Royal Chemical Society
- ^Moseley, H.G.J. (1913). 'XCIII.The high-frequency spectra of the elements'. Philosophical Magazine. Series 6. 26 (156): 1024. doi:10.1080/14786441308635052. Archived from the original on 22 January 2010.
- ^Eric Scerri, A tale of seven elements, (Oxford University Press 2013) ISBN978-0-19-539131-2, p.47
- ^Scerri chaps. 3–9 (one chapter per element)
- ^Ernest Rutherford | NZHistory.net.nz, New Zealand history online. Nzhistory.net.nz (19 October 1937). Retrieved on 2011-01-26.
- ^Origin of symbol Z. frostburg.edu
